INTRODUCTION
Amidst the COVID-19 pandemic and the dearth of antiviral agents without adverse effects, researchers have directed their efforts toward discovering novel antiviral compounds derived from natural origins (1). In recent years, scientific attention has been concentrated on scrutinizing natural products to unearth compounds suitable for antiviral drug development, aiming for minimal toxicity to humans (2). Subsequent synthetic campaigns have been undertaken to enhance the efficacy of these compounds against viral infections. Notably, herbal antiviral products have gained significant attention due to their resistance against viruses (3), escalating concerns regarding food safety, and the potential health ramifications associated with synthetic additives (4).
Artemisia, a noteworthy plant genus within the Asteraceae family, has gained considerable attention in scientific circles (5). This genus, commonly known as ‘Wormwood,’ ‘Mugwort,’ or ‘Tarragon,’ comprises over 500 species and commonly found in temperate regions across Asia, North America, and Europe, with Asia hosting the majority (6, 7). Several phytochemicals, including terpenoids, flavonoids, caffeoylquinic acids, coumarins, sterols, acetylenes, and volatile oils, are found in different Artemisia species (5). These plants exhibit diverse pharmacological applications, such as antimalarial, antihepatotoxic, cytotoxic, antifungal, antibacterial, antispasmodic, anti- diabetic, and antioxidant properties (8).
Artemisia has also shown potent antiviral activities against major viral infections. Thus, this review aims to explore the antiviral potential of Artemisia against viral diseases such as SARS-CoV-2, human and avian influenza viruses, human immunodeficiency virus (HIV), hepatitis B virus (HBV), and herpes simplex virus (HSV). The current research gaps, research opportunities, and future perspectives for this area of research are also provided.
Artemisia: AN OVERVIEW
The Artemisia genus encompasses various species, with some extensively utilized in traditional Chinese medicine for combating fungal, viral, and bacterial infections (Table 1) (9, 10). A notable compound, artemisinin, derived from Artemisia annua, belongs to the endoperoxide sesquiterpene lactone category. Artemisinin serves as a pivotal component in artemisinin combination therapies (ACT), exhibiting antimalarial efficacy for Plasmodium falciparum’s intraerythrocytic forms. Its mechanism involves impeding the detoxification of hemodigestion products (11). Researchers have explored novel derivatives for their effectiveness against malaria and several infections (12).
Table 1.
Pharmaceutical applications of Artemisia species (6)
Certain members of the Artemisia genus, referred to as wormwoods, possess historical significance as medicinal plants (13). Artemisia campestris, commonly called field wormwood, underwent pharmacological reassessment (14). Traditionally utilized as an antiseptic (15), A. campestris found application in treating pleurisy, a condition arising from viral or bacterial infections (16). Additionally, indigenous North American communities employ extracts of A. campestris to alleviate common colds induced by coronavirus or rhinovirus, although the underlying mechanism and active compounds remain undisclosed (17). This plant is usually employed as an anti-helmintic and for addressing respiratory, cutaneous, and digestive disorders.
Numerous publications have explored cytogeography, taxonomical affiliations, bioactive compounds, and biological activities of these plants (18). These works highlight the role of essential oils and aqueous extracts, abundant in terpenoids and flavonoids, in driving biological activity (Table 1). Assessment of antioxidant and anti-inflammatory properties has been conducted using diverse in vitro and in vivo approaches, encompassing measurements of superoxide dismutase activity, glutathione levels, nitric oxide products, protein oxidation, and carrageenan-induced rat paw edema (19). This research has unveiled specific targets of these plant metabolites in human viral infections as discussed in the next section of this review.
ANTIVIRAL ACTIVITIES OF Artemisia
SARS-CoV-2
The global pandemic of SARS-CoV-2 (the etiologic agent of COVID-19) has infected and killed millions of people. COVID-19 usually affects the upper respiratory tract while the common symptoms include headache, myalgia, fever, dyspnea, cough, and fatigue (20).
Various phytochemicals derived from diverse Artemisia species demonstrate notable antiviral efficacy for SARS-CoV-2 (21). Employing computational methodologies, researchers explored the antiviral activities of artemisinin and its derivatives to physically interact with key COVID-19 targets, such as the main protease (MPro), the spike ectodomain structural protein, spike receptor-binding domain, and the spike glycoprotein (Fig. 1). This interaction aims to hinder SARS-CoV-2 from binding to ACE2 (22). ADMET analysis of artemisinin indicated favorable aqueous solubility, non-cytotoxicity, and effective permeability via blood-brain barrier, suggesting strong antiviral potential. Based on molecular docking, artemisinin revealed strong binding to all four target proteins, at times exhibiting superior binding modes compared to hydroxychloroquine (23). Consequently, artemisinin-based compounds emerge as promising compounds for advancing antiviral drug development for SARS-CoV-2 infection (Table 2).
Table 2.
Antiviral activities of Artemisia species against major viral diseases
Target Virus | Species/Compound | Experiment | Mode of Action | Reference |
---|---|---|---|---|
SARS-CoV-2 | Artemisinin and its derivatives | In silico | Inhibition of binding with ACE2 | (22) |
Artemisinin-based compounds | In vitro | influencing multiple essential metabolic pathways | (25) | |
Artemisinin-piperaquine blend | Clinical trial | Not determined | (26) | |
Artemisinin and its derivatives | Clinical trial | Suppression of TGF-β | (30) | |
Influenza Viruses | A. cina | In vitro | Virucidal effect | (34) |
A. scoparia | In vitro,In vivo | Disruption of virus replication, reduction of hemagglutinin and M2 proteins, and attenuation of neuraminidase activity | (35) | |
A. rupestris | In vitro | Not determined | (37) | |
A.annua | In vitro | Activation of IL-6 and TNF-α expression | (38) | |
HIV | A.annua | In vitro | Inhibition of replication | (41) |
A.campestris subsp. glutinosa | In vitro | Blocking of viral entry and inhibition of transcription | (15) | |
HBV | A.argyi | In vitro | Inhibition of replication and antigen secretion | (50) |
A.argyi essential oil | In vivo |
Inhibition of sphingolipid, NF-κB, and TNF signaling pathways | (51) | |
HSV | A. vulgaris | In vitro | Interacting directly with the virus | (59) |
A. argyi | In vitro | disruption of HSV-1 viral particle membranes, resulting in compromised viral attachment and penetration | (60) |
Researchers have demonstrated the effectiveness of extracts from various Artemisia species against SARS-CoV-2 (24). Recent findings suggest that artemisinin-based compounds (ARTs) hinder SARS-CoV-2 pathogenesis by influencing multiple essential metabolic pathways, rendering them promising compounds for SARS-CoV-2 antiviral drug development (25). These outcomes have prompted several groups to initiate clinical investigations, aiming to assess the efficacy of ARTs in treating individuals infected with SARS-CoV-2.
In a recently conducted randomized clinical investigation, 41 individuals with confirmed COVID-19 were stratified into two cohorts. The control group comprised 18 subjects, while the experimental group (n = 23) was administered a blend of artemisinin- piperaquine (AP). The administration of AP commenced with an initial dose of two tablets (artemisinin 125 mg and piperaquine 750 mg) on day one, followed by a daily maintenance dose of one tablet (artemisinin 62.5 mg and piperaquine 375 mg) for 6 days (26). Despite the study’s constrained sample size and structure, the encouraging safety profile and immune-modulating attributes position AP as a prospective pharmaceutical candidate for addressing SARS-CoV-2 infection (26).
Transforming Growth Factor-beta (TGF-β) is an essential player in regulating immune responses, exhibiting diverse effects on various immune cell types. During SARS-CoV-2 infection, edema, pulmonary fibrosis, and cytokine storm characterize the advanced stages of the disease. The virus induces an up-regulation of TGF-β expression, contributing to the observed cytokine storm and lung fibrosis (27, 28). Ongoing efforts aim to identify novel small molecules capable of effectively inhibiting TGF-β production. Artemisinin and its derivatives demonstrated TGF-β suppressive effects in various inflammatory disease models (29). A Phase IV study is currently assessing the efficacy and safety of ARTs combination in COVID-19 patients (30). Beyond its strong antiviral action, this formulation is anticipated to alleviate TGF-β-mediated inflammatory damage linked to viral sepsis and cytokine storm. Early findings indicate a significantly faster patient recovery along with a favorable safety profile (30). Hence, the suppression of TGF-β responses by ARTs emerges as a promising antiviral strategy, positioning them as strong candidates for SARS-CoV-2.
Human and Avian Influenza Viruses
Influenza, a respiratory infection affecting both upper and/or lower respiratory tracts, stems from diverse influenza viruses (IVs), constituting a significant portion of the Orthomyxoviridae family (31). The influenza virus family encompasses four genera: influenza A, B, C, and D. The global impact of influenza is profound, resulting in 290,000 - 650,000 annual mortalities. The escalating resistance and adverse effects of established antiviral classes on the gastrointestinal tract and central nervous system diminish their efficacy in managing infections in specific vulnerable cases (32, 33). Hence, the imperative need for innovative antiviral compounds to combat this pathogen.
In a recent investigation, the extract from santonica flowers (Artemisia cina) demonstrated notable anti-H5N1 efficacy (34). Validation of its anti-influenza potential involved assessing selected plant extracts against the seasonal human influenza A/H1N1 virus. The santonica flower extract exhibited strong anti-influenza activity, comparable to its effectiveness against influenza A/H5N1. Moreover, the elucidation of the mode of action revealed a virucidal effect against the aforementioned influenza strains. Delving deeper into the chemometric component, the primary constituent, santonin, underwent in vitro screening (34) (Table 2).
Artemisia scoparia, employed in traditional Chinese medicine for addressing fever, cough, and cold, exhibits inhibitory effects against diverse influenza virus strains. The antiviral properties of A. scoparia were determined by elucidating their mechanisms against the influenza virus using in vitro and in vivo investigations (35). Their findings revealed the extract’s effective disruption of influenza virus replication, reduction of hemagglutinin and M2 proteins, and attenuation of influenza virus neuraminidase activity (Fig. 2) (35).
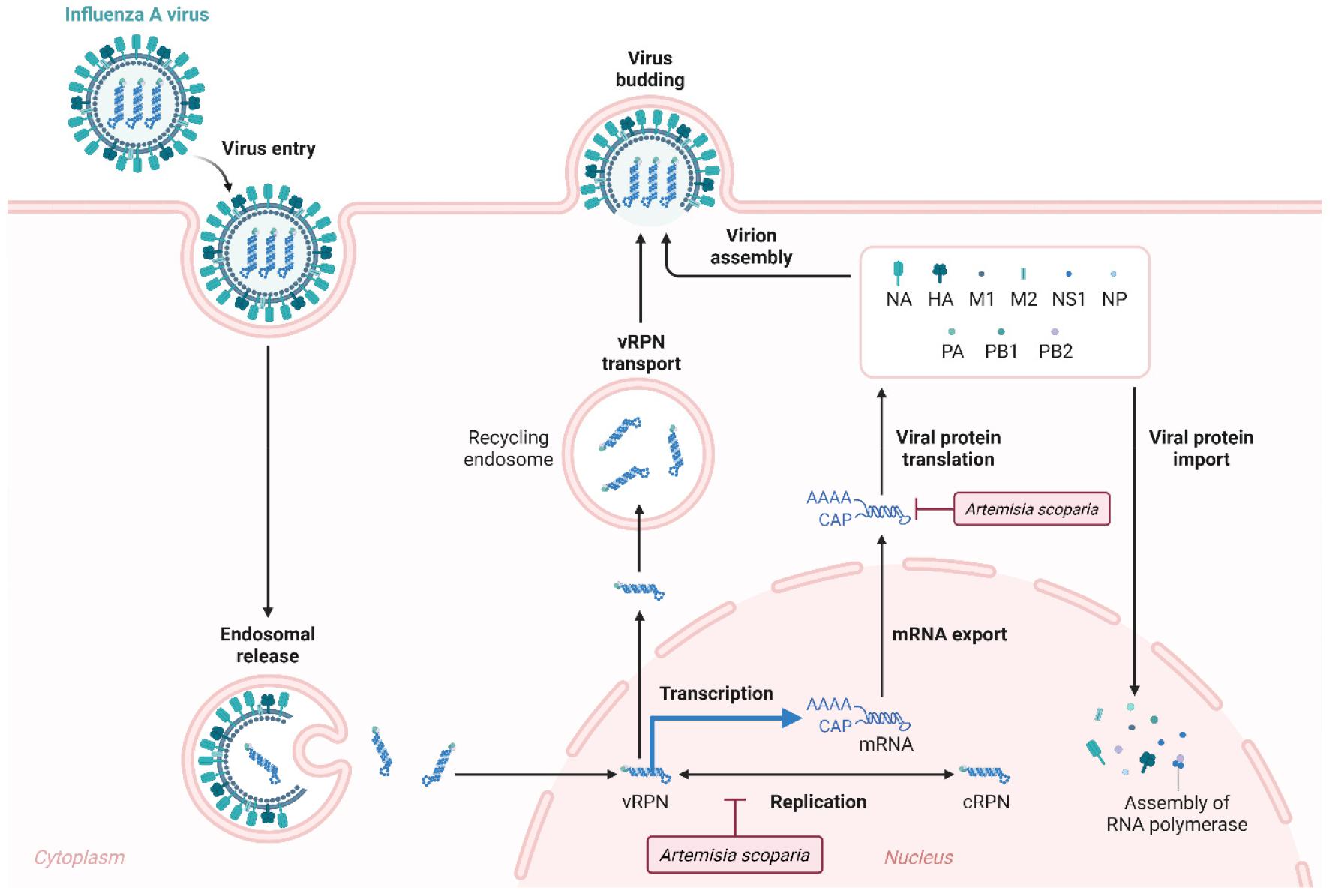
Fig. 2
Antiviral activity of Artemisia against influenza A virus. A. scoparia inhibits influenza viruses by causing the disruption of virus replication, reduction of hemagglutinin and M2 proteins, and attenuation of neuraminidase activity (35).
Artemisia rupestris L. is a perennial herb found in central Asia and China, and is traditionally utilized for treating colds (36). The aromatic and fatty ester derivatives of rupestonic acid, extracted from A. rupestris, exhibit notable antiviral properties against influenza A3 and B strains. The aromatic ester compound, in particular, demonstrated the strongest inhibitory activity against influenza A3 virus, nearly 10 times more potent than the reference drug oseltamivir (37).
Artemisia annua L. a medicinal plant sourced from Europe, Africa, and Asia, is utilized traditionally for addressing chills and fever (36). In Africa, it finds widespread cultivation for treating malarial fever (5). The impact of dihydroartemisenin from A. annua was investigated against the influenza A virus, revealing its capacity to inhibit IAV by activating interleukin 6 (IL-6) and tumor necrosis factor-α (TNF-α) expression. In the IAV group, TNF-α and IL-6 mRNA levels, along with TNF-α, IL-6, and phospho-ERK (p-ERK) protein levels, were strongly increased compared to the normal control group. Conversely, the IAV + dihydroartemisenin group reduced IL-6 and TNF-α mRNA levels, and TNF-α, IL-6, and p-ERK protein levels (38).
Human Immunodeficiency Virus
Human Immunodeficiency Virus (HIV) is a viral infection that impacts the immune system, eventually resulting in AIDS. This epidemic has impacted a significant number, with a considerable portion succumbing to complications related to AIDS (39). HIV, classified as a retrovirus in the Lentivirus genus, possesses a spherical structure measuring 100 nm in diameter. It carries two copies of single-stranded RNA and has the capability to transcribe within the host (40). Certain compounds derived from Artemisia exhibit promising anti-HIV activity.
An in vitro investigation revealed the anti-HIV potential of Artemisia annua tea. Given the prevalence of HIV-malaria coinfection in endemic regions, the inhibitory potential of Artemisia afra and Artemisia annua against HIV has been studied. Contrary to initial assumptions, artemisinin was not identified as the primary compound responsible for its anti-HIV effects. Notably, Artemisia annua tea infusion exhibited high inhibitory activity, whereas artemisinin displayed weaker inhibitory activity (Fig. 3) (39). In vitro models demonstrated a 60% inhibition of HIV-1 replication with 10 µM of artemisinin (41). Furthermore, 1,5-disubstituted 1,2,3-triazole dihydroartemisinin derivatives by Jana et al. (42) exhibited substantial inhibitory activity against HIV, whereas Effert et al. (43) reported lower inhibitory activities against two HIV-1 strains (Table 2).
Recent studies have also revealed that both aqueous and ethanolic extracts from Artemisia campestris subsp. glutinosa (Besser) Batt. subsp. glutinosa exhibit in vitro inhibitory activity against HIV, with the ethanolic extract demonstrating greater potency (15). Bio-guided fractionation of the ethanolic extract resulted in the identification of two terpenes: canrenone and damsin, and four flavonoids: acerosin, xanthomicrol, cardamonin, and 6, 2′, 4′-trimethoxyflavone. These phytochemicals displayed in vitro inhibition of HIV-1. The mechanism of their anti-HIV action involves blocking viral entry and/or inhibiting transcription. This action is not correlated with antioxidant activity but is associated with interference with Sp1 and NF-κB, which are currently unaddressed by antiretroviral therapy (15).
Hepatitis B Virus
Hepatitis B virus (HBV) stands as a significant global contributor to chronic liver disease, impacting over 290 million individuals (44). Annually, close to 800,000 deaths result from complications such as hepatocellular carcinoma (HCC) and cirrhosis arising from chronic HBV disease. Global mortality due to viral hepatitis is on the rise, projected to surge over 60% in 2040. Notably, anti-HBV drugs significantly diminishes the risk of HCC, cirrhosis, and chronic liver disease development (45, 46). However, the use of these drugs is linked to unfavorable side effects, encompassing leukopenia, thrombocytopenia, and renal and bone toxicity (47). Consequently, there is a pressing need to develop novel antiviral drugs devoid of side effects to combat this pathogen.
Artemisia argyi H.Lév. & Vaniot is a recognized medicinal plant deployed for managing dysmenorrhea, abdominal pain, and inflammation (48). A study identified 69 compounds from A. argyi essential oil (AAEO), with prevalent sesquiterpenes, monoterpenes (1,8-cineole and α-terpineol), and a relatively modest presence of ketones, aldehydes, aromatic, and phenolic compounds (49). Additionally, the study assessed the anti-HBV potential of AAEO, revealing substantial inhibition of HBV DNA replication and HBV antigen secretion (Fig. 4) (Table 2) (50).
In a recent investigation, nanostructured lipid carriers (NLCs) loaded with Artemisia argyi essential oil (AAEO) were formulated using hot homogenization and ultrasonication (51). The AAEO-NLCs significantly hindered HBV replication in duck HBV (DHBV) DNA, exhibiting a minimal virologic rebound upon treatment cessation in DHBV-infected ducks. Additionally, AAEO-NLCs demonstrated more reduced viral DNA levels compared to AAEO suspension. Pharmacokinetic and liver distribution studies validated that NLCs elevated liver exposure and enhanced the oral bioavailability of AAEO. Using network pharmacology, potential mechanisms of AAEO against HBV were revealed to be linked to immune response-related signaling pathways, including sphingolipid, nuclear factor kappa B, and tumor necrosis factor signaling pathways (51).
Herpes Simplex Virus
Herpes simplex virus (HSV) is a common human pathogen in the Herpesvirus subfamily, encompasses two variants, HSV-1 and HSV-2. These viruses, characterized by a linear double-stranded DNA structure and a complex spherical arrangement of at least 18 proteins, pose a significant health concern (52). Published data indicates an infection rate of 60-95% for herpes simplex virus type I in adults, while herpes simplex virus type II affects 60% of individuals (53). HSV-1 is implicated in conditions such as cold sores, herpes simplex conjunctivitis, and herpes simplex virus keratitis (54). HSV-1, a prevalent agent inducing infections in the central nervous system, has the potential to instigate critical focal necrotizing encephalitis. This condition is recognized for its elevated clinical mortality and poor prognosis, frequently culminating in persistent neurological impairments (55).
Presently, clinical treatments commonly employ nucleosides and analogs like acyclovir (ACV) and valaciclovir (VCV) (56). However, these drugs fall short of completely eradicating the virus or preventing HSV recurrence, especially in the early infection stages. The uncontrollable and frequent use of antiviral drugs has prompted virus mutations, resulting in heightened resistance of HSV-1 (57, 58). Consequently, there is a growing urgency to investigate novel drugs with efficacy against HSV-1.
In a recent investigation, diverse A. vulgaris L. plants were gathered from Tangyin County, Henan Province (59). Subsequently, A. vulgaris L. leaf extracts underwent screening for anti-HSV-1 activity. The plaque reduction test revealed that the A. vulgaris L. crude extract (Fr.8.3) demonstrated inhibitory activity against HSV-1. This finding was further confirmed through assessment at the DNA, RNA, and protein levels. Mechanistic exploration indicated that Fr.8.3 achieved its anti-HSV-1 activity by interacting directly with the virus (Table 2) (59).
In a separate study, it was established that A. argyi leaves (AEE) exhibit robust antiviral efficacy against both conventional and acyclovir (ACV)-resistant HSV-1 strains (60). AEE also impeded the invasion of HSV-2, influenza virus, and rotavirus. Further analysis revealed AEE’s disruption of HSV-1 viral particle membranes, resulting in compromised viral attachment and penetration. Furthermore, a mass spectrometry assessment identified 12 primary constituents of AEE. Notably, two newly identified flavones, 3,7-dihydroxy-3′,4ʹ-dimethoxyflavone and deoxysappanone B 7,3ʹ-dimethyl ether, displayed superior binding affinity to glycoprotein gB. This suggests their potential role in virion inactivation. Consequently, A. argyi emerges as a crucial source of antiviral drugs, with AEE representing a promising novel agent against HSV-1 infection (60).
CONCLUSION
In summary, the extensive review of Artemisia’s antiviral potential against a spectrum of viruses, including SARS-CoV-2, human and avian influenza viruses, HIV, HBV, and HSV, underscores its significant role as a promising therapeutic resource. The diverse bioactive compounds present in various Artemisia species exhibit remarkable inhibitory effects on viral replication and infection across multiple stages of the viral life cycle.
Artemisia’s efficacy in combating viral diseases is supported by compelling evidence from in vitro and in vivo studies. The nuanced mechanisms of action, such as interference with viral entry, transcriptional inhibition, and modulation of host immune responses, contribute to its broad-spectrum antiviral activity. These findings reinforce the potential of Artemisia-derived compounds as valuable candidates for antiviral drug development.
Despite the wealth of research, certain gaps persist. Further elucidation of specific molecular interactions, optimization of compound formulations, and comprehensive understanding of Artemisia’s effects on host factors during viral infections are areas warranting focused investigation. Standardized methodologies and comparative studies across Artemisia species can enhance our comprehension of their differential antiviral activities.
Artemisia stands at the forefront of antiviral research, poised for continued exploration and innovation. Collaborative efforts between virologists, pharmacologists, and botanists are crucial for unlocking the full therapeutic potential of Artemisia against emerging and established viral threats. Clinical trials and translational research are pivotal steps toward establishing the safety and efficacy of Artemisia-derived compounds, paving the way for their integration into mainstream antiviral therapeutics.