INTRODUCTION
Consumption of polyunsaturated fatty acids (PUFAs), particularly eicosapentaenoic acid (EPA) and docosahexaenoic acid (DHA), is associated with a reduced risk of obesity (1), breast cancer (2), diabetes mellitus (3), cardiovascular disease (CVD) (4), and metabolic disorders (5). The beneficial effects of n-3 PUFAs are closely associated with their anti-inflammatory activity. Previous studies have demonstrated that n-3 PUFAs modulate the activation of immune cells from both the innate and adaptive immune systems via diverse mechanisms (6) Although the specific mechanisms by which n-3 PUFA regulate immune responses could differ among cell types, n-3 PUFAs are incorporated into the cellular membrane and alter membrane fluidity and lipid rafts (7). Based on extensive information gathered from human and animal studies, n-3 PUFAs supplementation is considered a promising therapeutic approach for inflammatory and autoimmune diseases (7).
PUFAs are highly unstable and easily oxidized at room temperature, which can result in wide variations in the experimental results. Moreover, in mammals, n-6 PUFAs cannot be transformed into n-3 PUFAs. Caenorhabditis elegans encodes the fat-1 gene, which is an n-3 desaturase capable of converting n-6 PUFAs to n-3 PUFAs by catalyzing the formation of a double bond in the hydrocarbon chain (8). Thus, researchers have generated fat-1 transgenic mice harboring a codon-optimized fat-1 gene downstream of the mammalian β-actin promoter, allowing for systemic expression (9). Compared with C57BL/6 (WT) mice, fat-1 transgenic mice exhibit high levels of n-3 PUFAs derived from n-6 PUFAs in their tissues (8). Ultimately, the use of fat-1 transgenic mice allows researchers to examine the physiological effects of n-3 PUFAs while avoiding issues associated with differences in PUFA manufacturers, preparation, storage conditions, and feeding duration and timing (10).
Previous studies have demonstrated that fat-1 transgenic mice are resistant to dextran sulfate sodium plus azoxymethane- induced colon carcinogenesis, resulting from attenuated nuclear factor kappa B (NF-κB) activation, nitric oxide synthase expression (11), CD4+ T cells, and MФ infiltration (6). In other disease models, fat-1 mice were resistant to seizures (12); showed reduced liver tumorigenesis via decreased TNF-α and cyclooxygenase-2 expression (13); and attenuated acute lung injury by reducing leukocyte and macrophage infiltration, inflammatory protein-2, and thromboxane B2 (14). In other studies, increased n-3 PUFA levels in fat-1 mice reduced systemic inflammation in acute pancreatitis and tissue fibrosis in chronic pancreatitis (15). More recently, we reported that fat-1 expression attenuates allergic airway hypersensitivity (16), rheumatoid arthritis (17), and atopic dermatitis (18), as well as lung pathophysiology caused by acute viral infection (19). Despite the importance of B cells in adaptive immune responses, most studies have focused on T cell responses, and the effect of n-3 PUFAs on T cell activation, differentiation, and migration has been demonstrated in fat-1 mice by several groups (20, 21, 22). To elucidate the effect of increased n-3 PUFA levels in B cells, we evaluated B cell responses using a fat-1 overexpression mouse model.
MATERIALS AND METHODS
Animals and diets
Six to seven weeks C57BL/6 male mice (mice were purchased from Samtako, Inc. (Osan-si, Gyeongi-do, Republic of Korea) and fat-1 mice were kindly provided by Dr. J. X. Kang (Harvard Medical School, Boston, MA, USA). Mice were maintained under 12 h light-dark cycles at 22-25 at with 45–55% humidity and liberal access to food and water. All animals were given a 5% corn oil diet (Cat. D10001, Research Diets, Inc., New Brunswick, NJ, USA) for 4 weeks before experimentation. All experiments were followed with guidance from the Animal Care and Use Committee (ACUC) of Jeonbuk National University (CBNU 2016-72).
Splenic B cell isolation and culture
B cells were isolated from purified splenocytes with RBC lysis buffer and a MagniSort B cell isolation kit (Cat. 8804-6827-74, eBioscience, CA, USA) according to the manufacturer protocol, and cultured in RPMI-1640 medium (Cat. SH30027.01, GE Healthcare Life Sciences, Uppsala, Sweden) supplemented with 10% heat-inactivated fetal bovine serum (HI-FBS; Cat. FBS-22A, Capricon Scientific, Ebsdorfergrund, Germany), 50 mM 2-mercaptoethanol (Cat. M3148, Sigma Aldrich, St. Louis, MO, USA), 10 mM 4-(2-hydroxyethyl)-1-piperazineethanesulfonic acid (HEPES, Cat. H0557, Sigma Aldrich), 1 mM sodium pyruvate (Cat. S8636, Sigma Aldrich), and 0.05 mg/mL gentamycin (Cat. G1379, Sigma Aldrich).
Proliferation, differentiation analysis
B cell proliferation, plasma cell differentiation were examined after stimulation with 500 ng/mL lipopolysaccharide (LPS, Cat. L2630, Sigma-Aldrich) and/or 20 ng/mL recombinant IL-4 (Cat. 574302, BioLegend, San Diego, CA, USA). To assess proliferation assay, B cells were stained with carboxyfluorescein succinimidyl ester (CFSE) and then cultured at 1.0 ´ 106 cells/well in media containing the indicated concentrations of LPS and/or IL-4. After 3 days, cells were washed with fluorescence-associated cell sorting (FACS) buffer and analyzed by BD Accuri C6 flow cytometer (BD Biosciences, California, USA).
Plasma cell differentiation were monitored in stimulated cells cultured for 5 days and then stained with PE-conjugated anti-CD138 or biotin-conjugated IgG1 (Cat. 406604, BioLegend), IgG2b (Cat. 406704, BioLegend), IgG2a (Cat. 407104, BioLegend), IgG3a (Cat. 407104, BioLegend) and IgM (Cat. 406504, BioLegend). Biotin-labeled antibodies were subsequently detected the following streptavidin-conjugated FITC (Cat. 11-4317-87, eBioscience), PerCP-Cyanine 5.5 (Cat. 45-4317-82, eBioscience), or APC (Cat. 17-4317-82, eBioscience).
Western blot analysis
B cell lysate were collected and analyzed with western blotting. Samples were separated by 7% or 10% of SDS-PAGE gel electrophoresis and blotted onto nitrocellulose (NC) membrane. (Cat. HATF00010, Merck Millipore Ltd., Darmstadt, Germany). The membranes were probed with indicated primary antibodies. After 4℃ overnight incubation, the membrane was incubated with HRP-conjugated anti-rabbit secondary antibodies for 1hr in RT and visualized by West Femto Maximum Sensitivity Substrate (Cat. NCI34095KR, Thermo Fisher Scientific Inc., MA, USA) or West save gold (Cat. LF-QC0103, YOUNG IN FROMTIER, Seoul, republic of Korea) and Luminescent Image Analyzer System (Cat. LAS-4000, GE healthcare Life science, Little Chalfont, Germany). For detection of mitogen activated protein kinase (MAP-kinase), NF-κB, B cell signaling pathway. Used primary antibodies from Cell Signaling Technology Inc. Phospho-MAPK family cell signaling pathway antibody Kit (Cat. 9910), MAPK Family cell signaling pathway antibody kit (Cat. 9926), NF-κB cell signaling pathway kit (Cat. 9936), B cell signaling pathway kit (Cat. 9768S), AKT antibody (Cat. 4691P) and phosphor AKT antibody (Cat. 4606P), actin (Cat. AB3280, abcam, MA, USA).
Enzyme-linked immunosorbent assay
Cytokine secretion was quantified by ELISA kits from eBioscience as follows: TNF-a (Cat. 88-7324-88), IFN-g (Cat. 88-7314-88), IL-17 (Cat. 88-770-88), IL-10 (Cat. 431412), IgG (Cat. 88-50400-88), IgG1 (Cat. 88-50410-88), IgG2a (Cat. 8-50420-88), IgG2b (Cat. 88-50430-88), IgG3 (Cat. 88-50440-88), IgA (Cat. 88-50450-88), IgM (Cat. 88-50470-88), and IgE (Cat. 88-50460-88). All assays were performed according to the manufacturer’s instructions.
RESULTS
Increased n-3 PUFA levels suppress B cell proliferation and cytokine secretion
To evaluate the effects of n-3 PUFAs on B cell responses in vitro, mice were fed 5% corn oil diet. Splenic B cells were then stimulated with lipopolysaccharide (LPS) in the presence or absence of IL-4 for 72 h. As shown in Fig. 1, B cells from fat-1 transgenic mice exhibited significantly decreased proliferation. With LPS stimulation, the proportion of proliferated cells in WT mice was over 80%, whereas in fat-1 mice, the proportion was around 60%. Also, after LPS plus IL-4 treatment, the proportion of proliferated cells in WT mice reached to 85% and that in fat-1 mice was around 80% (Fig. 1). Similarly, the secretion of IL-6, TNF-a, and IL-10 was significantly lower in B cells overexpressing fat-1 mice than in WT mice (Fig. 2), and no differences were noted between LPS only and LPS+IL-4 stimulation.
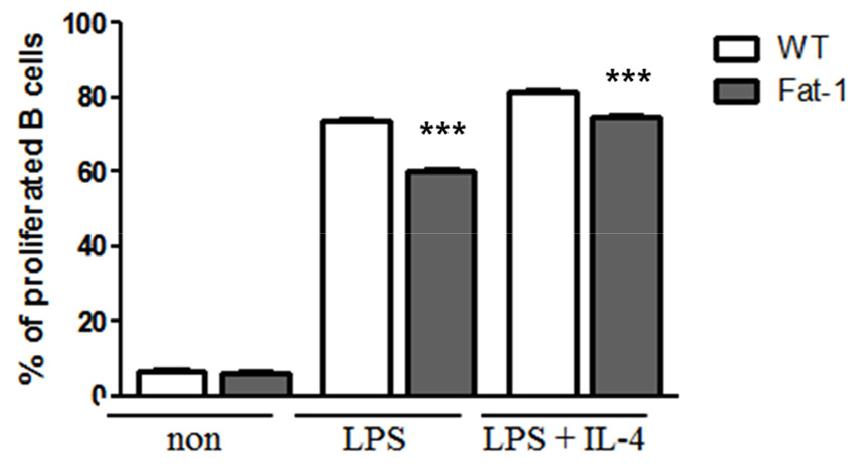
Fig. 1
Effect of increased n-3 PUFA on B cell proliferation. B cells were labeled with CFSE, cultured with the indicated stimulus for 72 h, and then analyzed by flow cytometer. Experiments were performed in triplicated and repeated twice independently. Data were shown as mean ± SEM. *** p<0.001 indicates statistical differences WT vs. fat-1 mice which were treated same stimulus.
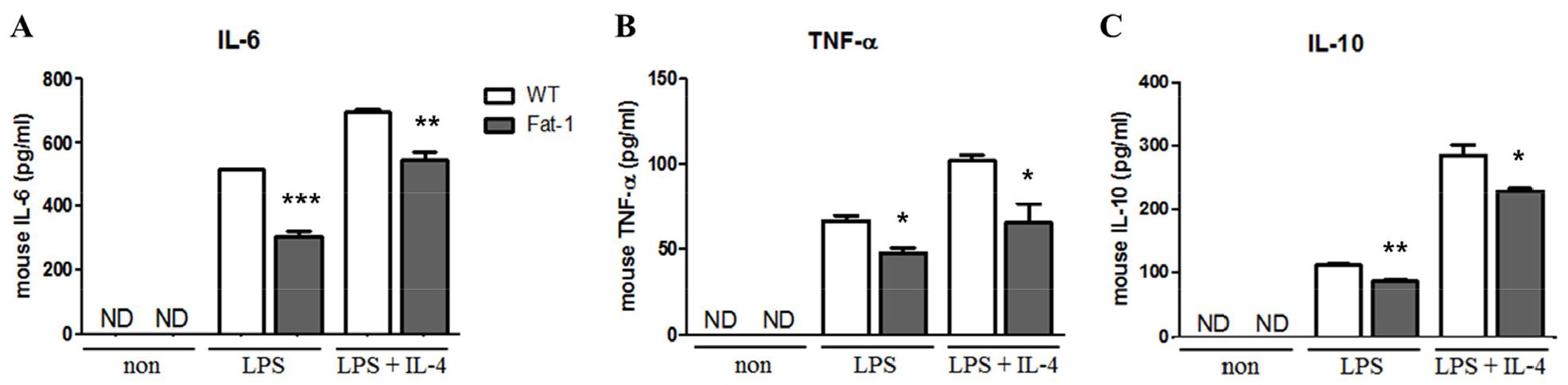
Fig. 2
Effect of increased n-3 PUFA on cytokine secretion by B cells. (A) IL-6, (B) TNF-α, and (C) IL-10 secretion was determined in cell culture supernatant from B cells treated with the indicated stimuli for 72 h. Experiments were performed in triplicated and repeated twice independently Data were shown as mean ± SEM. * p<0.05, ** p<0.01, *** p<0.001, indicates statistically differences WT vs. fat-1 mice which were treated with same stimulus.
Increased n-3 PUFA levels inhibit plasma cell differentiation, isotype switching, and antibody secretion
To determine whether fat-1 transgene expression altered plasma cell differentiation and antibody synthesis, B cells from WT or fat-1 mice were stimulated with LPS, with or without IL-4, for 5 days. Plasma cell differentiation was evaluated based on the expression of CD138, a plasma cell marker. Interestingly, fat-1 B cells showed a significant decrease in their differentiation into plasma cells (Fig. 3). When B cells from WT mice were stimulated with LPS or LPS+IL-4, the percentage of B cells differentiated into plasma cells was 10% or 17%, respectively. Increased n-3 PUFA levels in B cells via fat-1 overexpression remarkably reduced the ratio of plasma cells to 3% (LPS) or 8% (LPS+IL-4), respectively (Fig. 3). We also determined whether isotype switching was affected by increased n-3 PUFA levels in B cells. The ratio of IgG2a+, IgG2b+, and IgG3+ cells was significantly lower in B cells, which had increased n-3 PUFA levels via fat-1 overexpression, whereas the percentage of IgG1+ cells was comparable between WT and fat-1 B cells (Fig. 4). These results were subsequently confirmed using an antibody subclass-specific quantitative ELISA. When B cells were treated with LPS, the concentration of total IgG was lower in B cells from fat-1 mice; however, this difference was diminished when cells were stimulated with LPS+IL-4. IgG subclasses were differentially affected by increased n-3 PUFA levels in B cells (Fig. 5). While similar levels of IgG2b secretion were observed in B cells from WT and fat-1 mice, other subclasses, including IgG1, IgG2a, and IgG3, were secreted at much lower levels in B cells with fat-1 overexpression. Our results also demonstrated that isotype switching to IgA or IgE was suppressed by the transgenic expression of fat-1 in B cells. In summary, the results presented here provide further evidence that increased n-3 PUFA levels can downregulate B cell-mediated immune responses through the suppression of plasma cell differentiation, isotype switching, and antibody secretion.
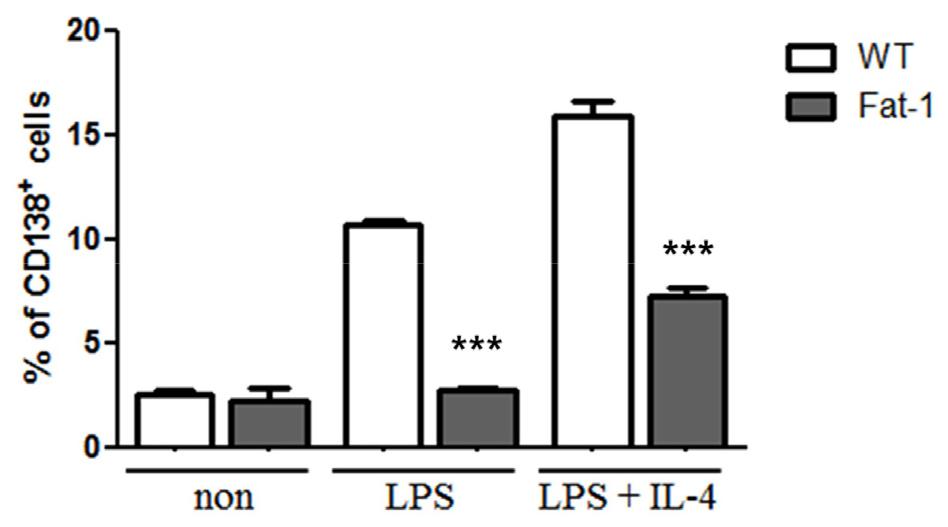
Fig. 3
Effect of increased n-3 PUFA on plasma cell differentiation. B cells were differentiated with the indicated stimulus for 5 days and then analyzed by flow cytometer. Data were represented by mean ± SEM from three independent experiments. *** p<0.001, indicates statistically differences between WT mice and fat-1 mice which were treated same stimulus.
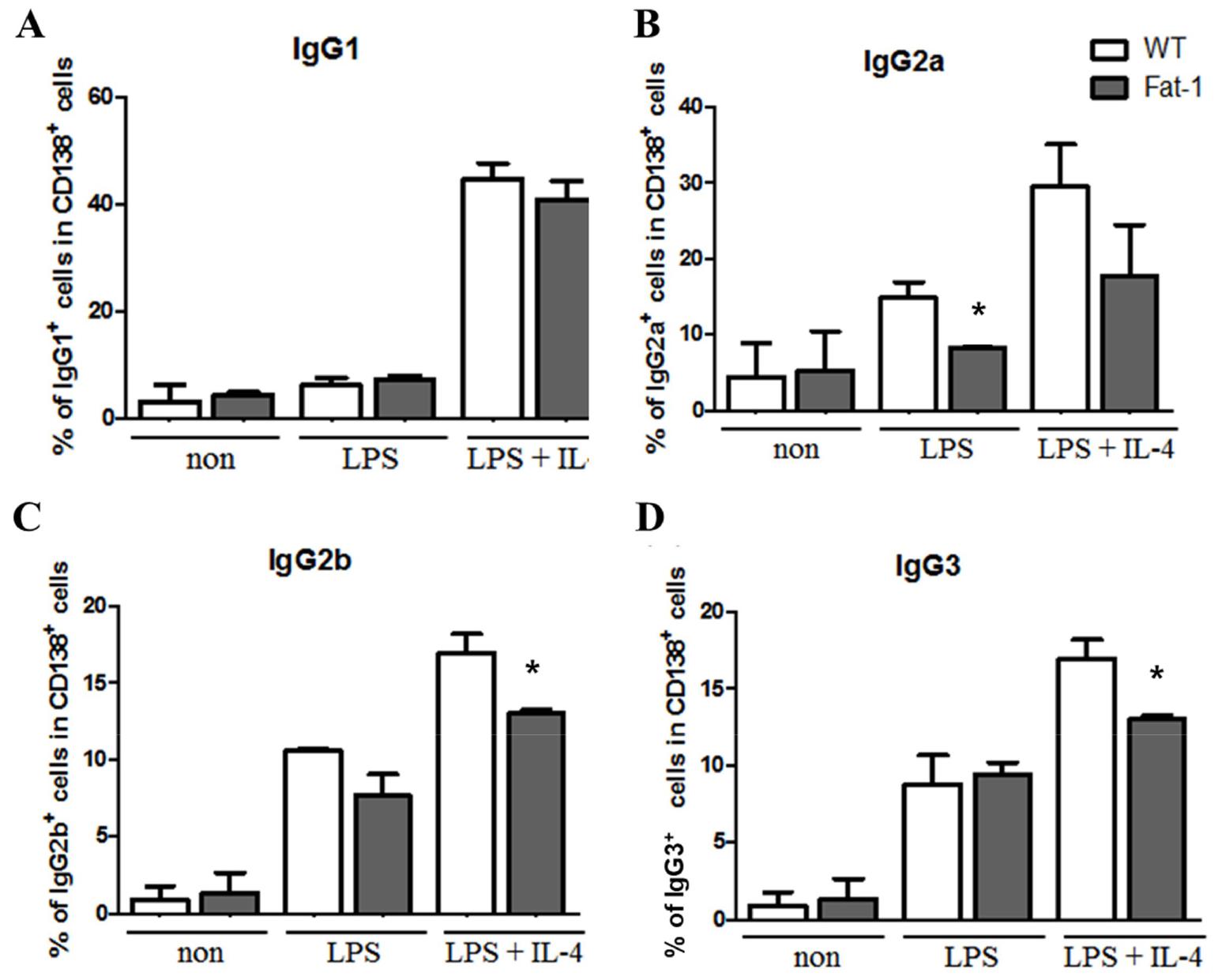
Fig. 4
Effect of increased n-3 PUFA on plasma cell surface Ig isotype expression. Surface Ig isotype expression was analyzed by flow cytometer. Experiments were performed in triplicated and data were shown as mean ± SEM of three independent experiments. * p<0.05, indicates statistically differences between WT mice and fat-1 mice which were treated with same stimulus.
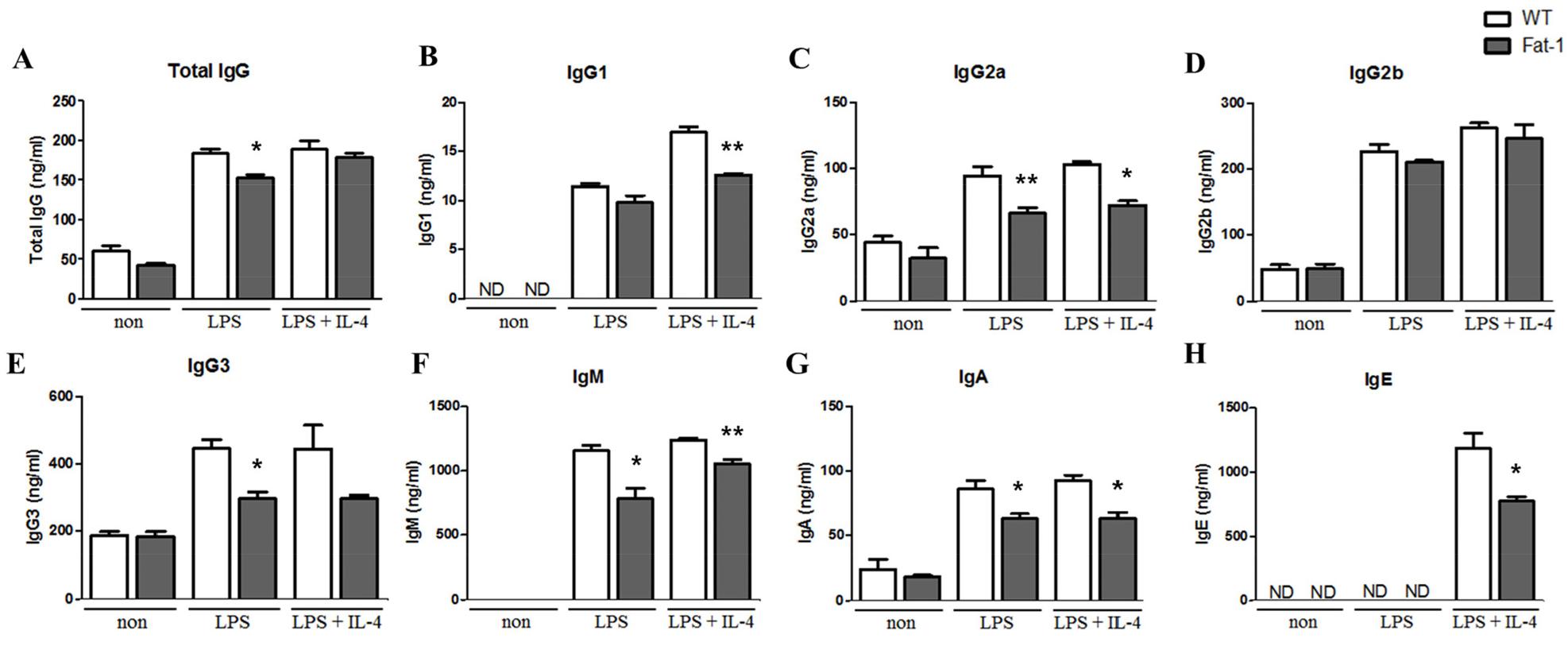
Fig. 5
Effect of increased n-3 PUFA on Ig secretion by plasma cells. Plasma cell Ig secretion was analyzed by ELISA. Experiments were performed in triplicated, and data were shown as mean ± SEM of three independent experiments. * p<0.05, ** p<0.01, indicates significant differences between WT mice and fat-1 mice which were treated with same stimulus.
Increased n-3 PUFA levels in B cells attenuate B cell receptor (BCR)- or CD40-mediated signal transduction
To explore whether higher levels of n-3 PUFA in B cells affect BCR- or CD40-mediated signaling pathways, purified B cells from WT or fat-1 mice were treated with either anti-F(ab’)2 or anti-CD40 antibody, and cell lysates were evaluated for the expression of signaling molecules using western blotting. Not surprisingly, phosphorylation of Syk, Src, and AKT was decreased in B cells overexpressing fat-1 upon BCR stimulation. In addition, CD40-mediated signal transduction was noticeably suppressed in B cells from fat-1 mice, as evidenced by the lower levels of pERK, pp65, and pAKT. (Fig. 6A/B). Taken together, these results indicate that increased n-3 PUFA levels may be responsible for reduced B cell activation, proliferation, and differentiation.
DISCUSSION
B cells play a major role in humoral immune responses, and antibody production is a hallmark of B cell function. Recent studies have demonstrated that B cells secrete various innate cytokines in response to pathogen-associated molecular patterns and contribute to inflammation (23). The production of autoantibodies and cytokines by dysfunctional B cells is increasingly recognized to play an important role in inflammatory and autoimmune diseases (24). B cell-targeted antibodies that deplete or reduce B cell numbers have been reported as promising therapeutics for rheumatoid arthritis, multiple sclerosis, and systemic lupus erythematosus (25).
In this study, we investigated whether increased n-3 PUFA levels affect B cell responses in fat-1 transgenic mice that are able to convert n-6 PUFA to n-3 PUFA in vivo. By using fat-1 mice, potential interferences with dietary compounding factors can be avoided. Previous studies have indicated that B cells respond differently to n-3 PUFAs (26). For instance, cells treated with palmitic acid or LPS exhibit decreased B220+ expression, whereas LPS- or BSA-treated cells display diminished IL-6 secretion. Alternatively, EPA and elaidic acid have no effect on B cell function (27). However, in another study, EPA and DHA enhanced the proliferation of Raji B cells (28). These results indicate that the effects of n-3 PUFA treatment can vary and may be cell type-dependent. Our results clearly demonstrated that increased n-3 PUFA levels via fat-1 transgene expression attenuate B cell proliferation and cytokine secretion compared with B cells isolated from WT mice.
Upon activation, B cells differentiate into plasmablasts, plasma cells, and memory cells. Plasma cells finally differentiate into short-lived and long-lived plasma cells, which secrete antigen-specific IgG (29). Antibodies can be classified into five isotypes (IgM, IgD, IgG, IgE, and IgA), and IgG isotypes are divided into five sub-isotypes (IgG1, IgG2a, IgG2b, IgG2c, and IgG3) (30). Plasma cells initially secrete IgM but undergo isotype switching to IgG, IgA, or IgE, the process of which is controlled by cytokines produced by helper T cells (31, 32). In a previous study, LA-, EPA-, and DHA-treated splenocytes isolated from Sprague-Dawley rats showed decreased IgM, IgG, and IgA production following lectin stimulation (8). Furthermore, diminished OVA-specific IgE and IgG1 secretion has been observed in mice fed a 25% fish oil diet, accompanied by decreased eosinophil and lymphocyte infiltration (33). Similarly, we found that fat-1 B cells were defective in plasma cell differentiation, Ig subtype switching, and Ig secretion, suggesting that fat-1 overexpression attenuates B cell responses. In the present study, the molecular and immunological mechanisms involved in these phenomena were not revealed, and further studies are required.
BCR and CD40 ligation with antigen and CD40 ligand (CD40L), respectively, lead to the activation of downstream NF-κB, MEK/ERK, and PI3K/AKT/mTOR pathways (34). Our results showed that increased n-3 PUFA levels in B cells suppressed BCR- and CD40-mediated signaling, resulting in lower levels of phosphorylated Syk, Src, ERK, AKT, and p65. Therefore, it is plausible that decreased BCR and CD40 signaling is responsible for reduced B cell proliferation and differentiation, as well as cytokine production of B cells from fat-1 mice. Several studies have demonstrated that n-3 PUFA treatment exerts anti-inflammatory activity via suppression of the NF-κB pathway; this was further confirmed by the identification of G-protein coupled receptor 120 (GPR120) as an n-3 PUFA receptor, which activates transforming growth factor-β activated kinase 1 (TAK1) and subsequently inhibits NF-κB (35, 36, 37).In addition to TAK1, the inhibition of STAT4 by increased n-3 PUFA levels is associated with reduced Th1 differentiation (38). The effect of n-3 PUFA on BCR signaling has been reported in a few studies. Ramon et al reported that DHA treatment inhibited IgE production by human primary B cells via direct interference with STAT6 and NF-κB (39). Although the present study shows that the activation of upstream signaling molecules of BCR is partially blocked by n-3 PUFA, further studies are necessary to fully elucidate molecular mechanisms by which enhanced n-3 PUFAs suppress B cell responses.
Collectively, we demonstrated that enhanced n-3 PUFA levels in B cells from fat-1 B cells negatively regulate overall B cell responses, including proliferation, cytokine secretion, plasma cell differentiation, isotype switching, and Ig secretion, possibly through decreased lipid raft clustering and subsequent attenuation of BCR- and CD40-mediated signal transduction. Our findings suggest that increased n-3 PUFA levels may be helpful in treating or preventing various autoimmune or inflammatory diseases mediated by autoantibodies, as well as allergic diseases caused by IgE overproduction.