INTRODUCTION
The global incidence of NTM lung disease is a growing public health concern on a global scale. While more than 180 species have been identified to date, only a few are known to cause disease (1). Among human pathogens, Mycobacterium avium complex (MAC) and Mycobacterium abscessus species (MAB) are the most frequent pathogens causes of pulmonary disease worldwide (2). It has been determined that MAC is the most common causative agent of NTM disease; however, infections caused by MAB are notoriously challenging to treat due to their high inherent resistance to many antibiotics, including anti-TB drugs, such as rifampin (3). Furthermore, M. abscessus exhibits resistance to macrolide antibiotics, such as clarithromycin (CLR) and azithromycin (AZM), as well as amikacin, which is frequently used to treat M. abscessus infections (4, 5). However, despite drug resistance issues, CLR remains the most effective drug against M. abscessus infection (6). In general, long-term therapy with multiple antimicrobial drugs is usually required for treatment of M. abscessus infection. According to current guidelines, a combination therapy of macrolide with intravenous agents is generally recommended for a period of at least two weeks to several months (7). M. abscessus infection is typically difficult to treat even with prolonged antibiotic treatment and lung transplantation may not be a good treatment alternative due to severe complications (8, 9). Consequently, the development of novel antimicrobial agents is a critical strategy for managing M. abscessus infections.
Acetohydroxyacid synthase (AHAS) is the pivotal enzyme implicated in biosynthesis pathway for the branched-chain amino acids (BCAAs) including isoleucine, leucine, and valine. This enzyme has been extensively characterized in bacteria, plants, and fungi (10). AHAS is found in microorganisms and plants, but not in animals (11, 12). It is widely acknowledged that five distinct classes of AHAS inhibitors, namely sulfonylurea (SU), imidazolinone (IMI), triazolopyrimidine (TP), pyrimidinyl-benzoate (PYB), and sulfonylamino-cabonyl-triazolinone (SCT), have been utilized as herbicides over three decades (13). In the last few years, there has been a surge of interest in the development of novel antimicrobial agents targeting AHAS. In environments where amino acid availability is limited, intracellular pathogens such as Mtb can utilize the second supply of amino acids, including the BCAA biosynthesis pathway, for replication (14). The BCAA biosynthesis pathway is a vital nutrient for Mtb growth and survival (15). Previous studies have demonstrated that AHAS inhibitors, sulfometuron methyl, exhibit in vitro anti-TB activity (16, 17). Consequently, the development of novel AHAS inhibitors with robust antimycobacterial activity has emerged as a promising target for the treatment of NTM, including drug-resistant NTM. In our previous study, we reported the antimicrobial and synergistic effects of the AHAS inhibitor K13787 in combination with CLR against M. abscessusin vitro. The present study was conducted with the objective of investigating the inhibitory effect of K13787 in amouse infection model. The findings of this study suggest that the inhibition of BCAA biosynthesis through the targeting AHAS could be a novel strategy for the development of antimicrobial drugs as a candidate for pan-mycobacterial drugs including M. abscessus.
METHODS
Triazolopyrimidine-2-sulfonamide ASAH inhibitors
Triazolopyrimidine-2-sulfonamide ASAH inhibitor, K13787 was synthesized in the Korea Research Institute of Chemical Technology (Daejeon, South Korea). The general procedures for synthesis of triazolopyrimidine-2-sulfonamide are described elsewhere (18, 19). To a solution of sulfonyl chloride 1 (0.11 mmol) and aniline 2 (0.17 mmol, 1.5eq) in anhydrous acetonitrile (1 ml) was added 3, 5-lutidine (0.33 mmol, 3eq). The reaction mixture was stirred at 40℃ for 3 h and subsequently poured into aq. HCl solution (0.1N, 10 ml). The product was extracted with ethyl acetate (2×10 ml) and dried over anhydrous Na2SO4. The organic phase was concentrated in a vacuum apparatus to yield the crude mixture, which was subsequently purified by column chromatography (using a mixture of 10% ethyl acetate and hexane) to afford K13787 (20).
Cell cultures
To obtain the required sample, murine bone marrow-derived macrophages (BMDMs) were extracted from femurs and tibias of C57BL/6 mice (6–9 weeks of age). The differentiation of BMDMs was then induced through a 4–5 day growth phase in Dulbecco’s modified Eagle medium (DMEM), which was enriched with the macrophage colony-stimulating factor (M-CSF, 25 µg/ml; R&D Systems, Minneapolis, MN, USA). This phase was conducted at 37℃ and 5% CO2 to unsure optimal differentiation of the cells.
Bacteria culture
Mtb strain H37Rv (ATCC 25177) and M. abscessus (ATCC 19977) was purchased from the American Type Culture Collection and cultured in Middlebrook 7H9 liquid medium containing 10% OACD (oleic acid, albumin, dextrose, and catalase), combined with 5% glycerol. Bacterial cultures were grown at 37℃ with shaking at low speed (140 rpm) until they reached the log phase (OD600 = 0.6–1). The bacteria samples were harvested by means of centrifugation at 3,000 × g for a period of 30 min. Thereafter, the samples were washed thrice with phosphate-buffered saline (PBS). Following this, the bacteria were resuspended in PBS, and aliquots of the bacterial suspension were stored in a -70℃ freezer until use. The thawed bacterial aliquots were dispersed using a bath sonicator (a few seconds) and then used for testing of antimycobacterial activity and infection. To determine colony-forming units (CFU)/ml, dispersed bacteria were prepared by serial dilution in 7H9 medium and plated on 7H10 agar. All NTM species met the criteria of the “Clinical and Laboratory Standards Institute (CLSI M24) (21).”
Intracellular survival analysis
The intracellular survival of M. abscessus in BMDMs was quantitatively analyzed by CFU analysis. BMDMs were infected with M. abscessus at multiplicity of infection (MOI) of 5 for a period of 3 h. To remove non-phagocytized bacteria, the cells were washed with PBS, and the cells were further incubated at 37℃ in 5% CO2 for 48 h in fresh medium without antibiotics. The cells were then lysed in 1 ml of autoclaved distilled water per well to collect intracellular bacteria. The lysates were plated separately on 7H10 agar plates and incubated at 37℃ for 3 days for M. abscessus.
In vivo model
In this study, C57BL/6 WT mice (female, 6 weeks of age) were utilized in this study and housed under specific pathogen-free conditions. The WT mice were purchased from NaraBiotech (Seoul, South Korea) and subsequently infected intratracheally with M. abscessus at a concentration of 1.4 x 107 CFU. The negative control group received a 2% polyethylene glycol (PEG) solution, the positive control group received CLR (200 mg/kg), and the experimental group received K13787 (200 mg/kg). CLR and K13787 were dissolved in 2% PEG for drug administration. For the assessment of bacterial loads, CLR and K13787 were administered intraperitoneally at 24 and 48 h, and then on the fourth day, the mice were euthanized, and the bacterial loads in the lungs and spleen were analyzed by CFU assays on 7H10 plates. The data are expressed as the mean ± standard deviation (SD) (n = 9, 3 mice/each experiment) and are pooled from three independent experiments. To assess survival rate, CLR and K13787 were administered intraperitoneally or orally at 24 and 48 h, and the survival of the mice was subsequently recorded for an extended period. The data are presented as the mean ± SD (n = 45, 15 mice/group) and are pooled from three independent experiments.
Statistical analysis
For the purpose of conducting statistical analyses, the data obtained from independent experiments are presented as means ± SD. It is imperative to note that all experiments were performed independently and repeated a minimum of three times. Subsequently, statistical analysis was performed using one-way analysis of variance (ANOVA) followed by Dunnett multiple comparisons test between groups. This analysis was conducted using GraphPad Prism Software (version 8.0; GraphPad Software, San Diego, CA, USA). Statistically significant differences were considered as those with values of *p < 0.05, **p < 0.01 and ***p < 0.001.
RESULTS
K13787, an inhibitor of the AHAS, regulates the growth of mycobacteria in BMDMs
Previous studies have identified sulfonylurea analogues with antibacterial activity against NTM and Mtb (22). Recently, it was reported that K13787, a pyrazolopyrimidine sulfonamide AHAS inhibitor, exhibited inhibitory effects against M.abscessus and M. avium(20). However, the in vivo activity of K13787 against M. abscessus has not yet been investigated. In this study, we further examined the inhibitory capacity of K13787 against M. abscessus using mouse infection model. The chemical structure of K13787 was shown in Fig. 1A.
Firstly, the inhibitory effect of K13787 on the intracellular growth of M. abscessus in BMDMs following infection was assessed. Treatment with K13787 resulted in a significant reduction in the intracellular growth of M. abscessus at 24 h and 48 h in BMDMs in comparison to the untreated control. However, the activity of K13787 was found to be less effective than that of CLR, which was used as a positive control (Fig. 1B). Furthermore, K13787 exhibited suppressive activity against M. tuberculosis (Table 1). Collectively, these findings indicate that K13787 has the potential to be a pan-mycobacterial drug candidate.
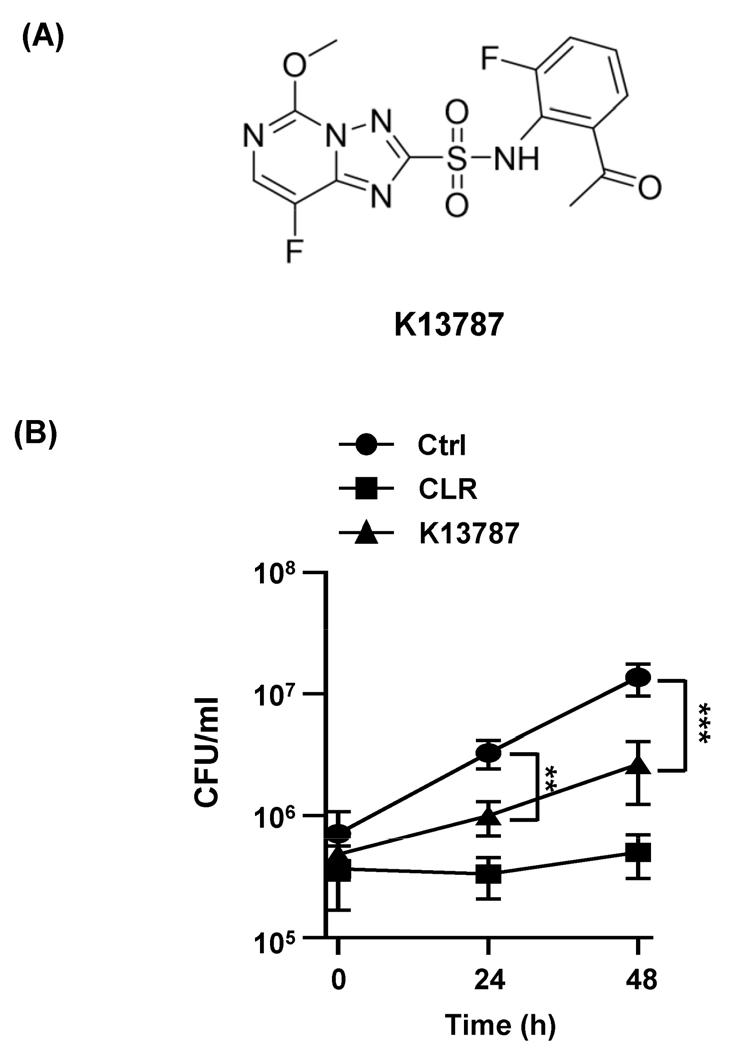
Fig. 1
K13787 inhibit the growth of M. abscessusin vitro. (A) The chemical structure of K13787. (B) BMDM cells were infected with M. abscessus (MOI = 5) and then treat with CLR (50 µg/ml) or K13787 (50 µg/ml) for 48 h. Intracellular survival was assayed by CFU assays on 7H10 plates. Data are shown as mean ± SD of three independent experiments. **p < 0.01, and ***p < 0.001
Table 1.
MIC of K13787 against M. tuberculosis.
Strain | MIC (µg/ml) | ||
---|---|---|---|
INH | RIF | K13787 | |
M. tuberculosis | 0.06 | 0.13 | 0.24 |
K13787 has an inhibitory effect on the growth of M.abscessus in vivo
To assess the inhibitory efficacy of K13787 in vivo, mice were infected with M. abscessus (1.4 x 107 CFU) and subsequently treated with K13787 or CLR via intraperitoneal or oral administration twice at 24- and 48-h post-infection (Fig. 2A). Four days post-infection, bacterial load was evaluated in the lung and spleen. As illustrated in Fig. 2B and 2C, K13787 treatment led to a substantial decrease in bacterial loads in both tissues, similar to the effects observed with CLR injection. Moreover, K13787 administration resulted in a notable enhancement in survival rates among M. abscessus-infected mice, irrespective of the delivery route (intraperitoneal or oral) (see Fig. 2D and 2E). While the antimicrobial activity of CLR was higher than that of K13787 in vivo, K13787 still exhibited significant efficacy in reducing bacterial load in tissues and improving survival rates. Collectively, these finding indicated that K13787 has potential as a lead compound for the future development of antimycobacterial drugs against M. abscessus infection.
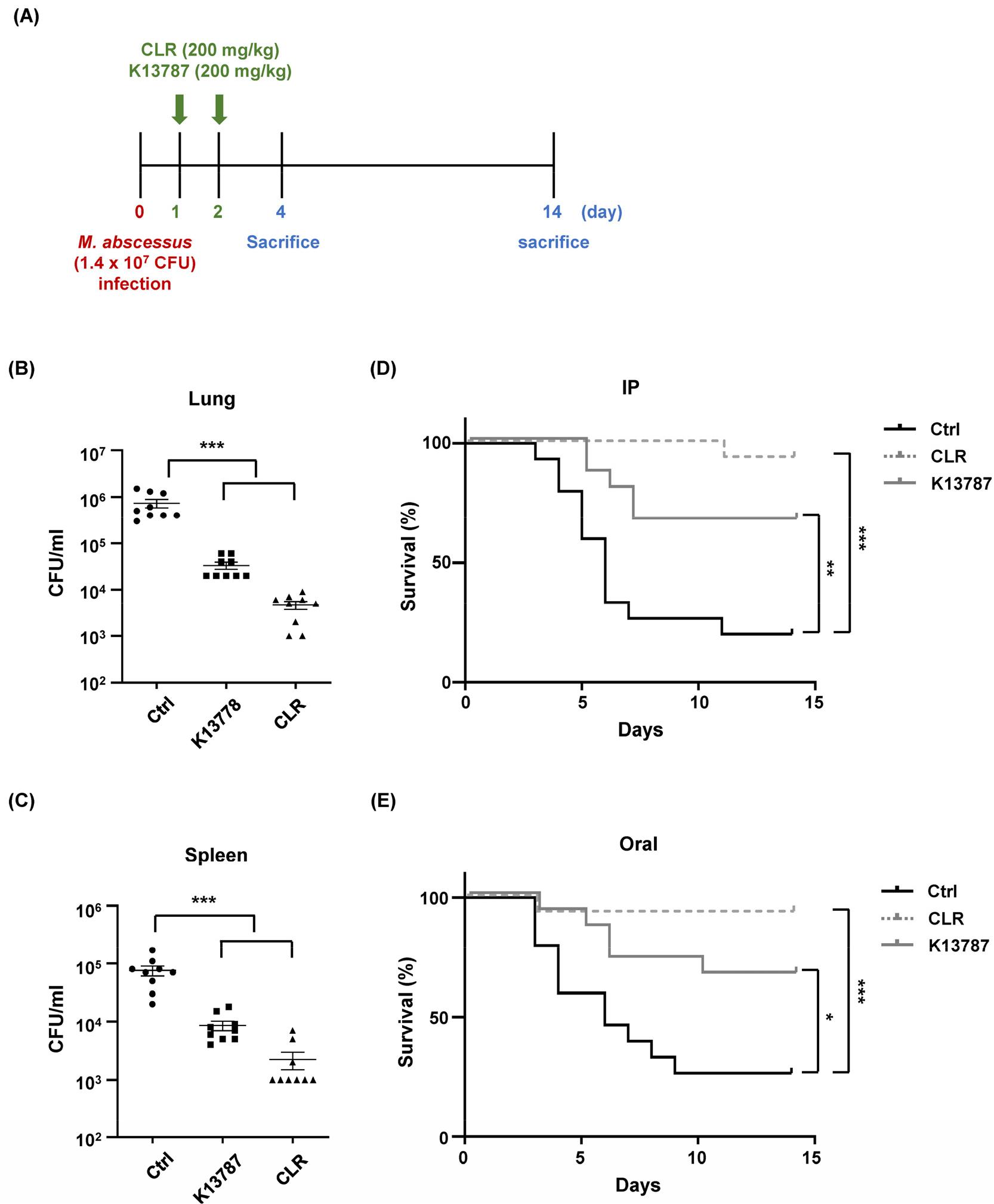
Fig. 2
K13787 treatment improve survival of M. abscessus-infected mice. (A) Schematic diagram of the in vivo experimental procedure for treatment with CLR and K13787 under M. abscessus infection. (B, C) WT mice were infected with M. abscessus (1.4 x 107 CFU) for 4 days. The negative control group received 2% polyethylene glycol (PEG) solution, the positive control group received CLR (200 mg/kg), and the experimental group received K13787 (200 mg/kg). CLR and K13787 were dissolved in 2% PEG for drug administration and were intraperitoneally administered at 24 h and 48 h. On the fourth day, the mice were sacrificed, and bacterial loads in the lungs and spleen were analyzed by CFU assays on 7H10 plates. Data are shown as mean ± SD (n = 9, 3 mice/ each experiment) and are pooled from three independent experiments. (D, E) WT mice (n = 45, 15 mice/group) were infected with M. abscessus (1.4 x 107 CFU) for 2 weeks. The negative control group received 2% PEG solution, the positive control group received CLR (200 mg/kg), and the experimental group received K13787 (200 mg/kg). The first experimental group received intraperitoneal (IP) administration, while the second experimental group received oral administration. The survival of the mice was recorded and illustrated daily for 2 weeks after infection. *p < 0.05, **p < 0.01, and ***p < 0.001
DISCUSSION
The anti-bacterial effect of sulfonylurea class as an AHAS inhibitor is well documented (23, 24, 25, 26). Our previous study demonstrated that AHAS inhibitors including sulfonylurea group, exhibited antimycobacterial activity against Mtb and M. abscessus (22). Furthermore, we reported that K13787, a triazolopyrimidine sulfonamide derivative targeting AHAS, exhibited a synergistic effect with CLR against M. abscessus and M. avium(20). However, the antimicrobial effect of K13787 has not yet been examined in vivo. In the present study, we found that K13787 significantly suppressed the growth of M. abscessus in both in vitro and in vivo settings. Treatment with K13787 led to a significant reduction in the intracellular survival of M. abscessus in mouse tissues such as the lung and spleen, and in BMDMs compared to the control. Furthermore, intraperitoneal and oral administration of K13787 to mice infected with M. abscessus resulted in a substantial improvement in survival rates (Fig. 3).
BCAA biosynthesis plays pivotal role in the survival of intracellular bacterial by supplying amino acids for protein synthesis in nutrient-deprived environments and maintaining central metabolism in bacteria (10, 27). Given that mycobacteriaare known to replicate within professional phagocytes, where amino acid availability is limited, it has been posited that the inhibition of AHAS may emerge as a promising strategy for the development of antimicrobial agents (28). Recent research has demonstrated that MRSA and colistin-resistant E. coli exhibit increased BCAA consumption compared to non-resistant strains (29). Novel AHAS inhibitors have also demonstrated anti-TB activity including MDR and XDR isolates in vitro(16). Consequently, AHAS inhibitors, exemplified by K13787, emerge as promising therapeutic candidates for combating drug resistant bacterial strains, including CLR-resistant M. abscessus.
Furthermore, the inhibition of BCAA by sulfonylurea-targeted AHAS has demonstrated the growth of Mtb and Brucella suis is suppressed in macrophages (17, 30). Sulfonamide constitutes on of the primary structures of AHAS inhibitors, including K13787. Sulfonamides exhibit a variety of biological activities, including antibacterial (31, 32), antiviral (33), antifungal (34), and antiprotozoal (35, 36) properties. The structural similarity between sulfonamide and p-aminobenzoic acid (PABA), which is essential for DNA production in bacteria (37), suggesting a potential mechanism by which sulfonamide can regulate bacterial DNA growth and cell division. This regulatory effect occurs through the interference with tetrahydrofolate synthesis by inhibiting and substituting PABA in the enzyme dihydropteroate synthetase (38). Our findings suggest that K13787 may impact the DNA production of M. abscessus, leading to its loss within cells.
Taken together, these results suggest that novel AHAS-targeted compounds such as K13787, which contain a sulfonamide group, have the potential to be used as pan-mycobacterial drugs for the treatment of intractable TB as well as NTM infectious disease in the future.
ABBREVIATIONS
NTM, nontuberculous mycobacteria; TB, tuberculosis; MOI, multiplicity of infection; CFU, colony-forming units; MAB, M. abscessus species; Mtb, Mycobacteria tuberculosis; AHAS, acetohydroxyacid Synthase, minimum bactericidal concentration; M-CSF, macrophage colony-stimulating factor; BMDMs, bone marrow-derived macrophages; WT, wild-type; PBS, phosphate-buffered saline; CLR, clarithromycin.